Description:
A device and method for path-integrated optical sensing and simultaneous retroreflector tracking using a multi-functional position-sensing photodetector
Princeton Docket # 11-2700-1
Detection of molecular species is of importance in a number of industrial, health and atmospheric monitoring applications. Existing spectroscopic gas sensing instrumentation has some fundamental limitations, primary due to the fact that signals carrying the useful information are encoded in light intensity, thus the measurements are strongly affected by fluctuations of the laser power reaching the photodetector. Moreover, due to exponential character of the absorption process (saturation-like effect), the performance of commonly used methods that rely on measuring the absorption caused by the target chemical species deteriorates when the peak absorption increases above 10-20 %. In order to improve the capabilities of non-invasive laser-based chemical sensing we have developed a chirped laser dispersion spectroscopy (CLaDS) ¿ a sensitive, multi-species sensing technology. In contrast to commonly used absorption methods, CLaDS detects molecular concentration by measuring optical dispersion, which provides several useful advantages. CLaDS dispersion signal is encoded in frequency of the measured RF signals. Thus one of the key capabilities of CLaDS is its immunity to detected laser power fluctuations that is similar to well established FM radio communication. Moreover, since the dispersion signal is linear with concentration of the target analyte, CLaDS is capable of precise sensing in a much wider concentration range (from the minimum detection limit to nearly 100% absorption). Those key CLaDS capabilities are further enhanced through an application of a new chirp modulated CLaDS (CM-CLaDS) that enables improved detection limits and provides true baseline-free spectroscopic detection. These properties make CM-CLaDS suitable for a wide range of sensitive spectroscopic chemical detection applications in both single-point extractive sensing as well as in open-path remote sensing configuration.
APPLICATIONS
|
ADVANTAGES
|
- Environmental Gas Monitoring: Detection and quantification of trace gases (e.g., greenhouse gases like N2O, CO2, CH4)
- Open Path Remote Sensing for atmospheric pollutant measurements over long distances.
- Industrial Process Control: Real-time monitoring of gas concentrations in manufacturing and chemical processes to ensure safety, quality, and efficiency.
- Medical Diagnostics: Non-invasive breath analysis for detecting biomarkers and monitoring patient health.
- Scientific Research: Laboratory studies requiring sensitive, baseline-free molecular detection, including spectroscopy and physical chemistry experiments.
- Security and Defense: Standoff detection of hazardous chemicals or explosives in security and defense applications.
- Single-Point and Distributed Sensing: Can be used in both extractive (single-point) sensors and distributed open-path sensor networks.
|
- Baseline-Free Detection: The 2nd harmonic (2f) signal analysis eliminates baseline artifacts caused by interferometer path imbalance, providing accurate, drift-free measurements.
- Reduced Noise and Improved Sensitivity: Narrowed acquisition bandwidth and harmonic filtering reduce frequency demodulation noise, resulting in up to 3× sensitivity improvement over conventional CLaDS, with potential for 10–100× improvement as optical fringe noise is further suppressed.
- Immunity to Optical Power Variations: Maintains signal amplitude and signal-to-noise ratio even with significant changes (up to 20 dB) in optical power, making it robust for field and open-path applications.
- Real-Time Calibration: The 1st harmonic (1f) signal enables real-time determination of laser chirp rate and modulation depth, simplifying calibration and ensuring measurement accuracy.
- Continuous and Versatile Monitoring: Supports both frequency scanning for spectral analysis and laser locking for continuous, real-time concentration monitoring.
- Easy Integration: Can be implemented in existing CLaDS and conventional laser absorption systems without major hardware modifications, facilitating rapid adoption.
- Large Dynamic Range:
- Provides linear response from trace levels (ppb) up to near-saturation concentrations, outperforming traditional absorption-based techniques.
- Enhanced Robustness: More immune to opto-mechanical fluctuations and environmental disturbances compared to conventional methods
|
Fundamentals of CLaDS
CLaDS is based on measurement of optical dispersion associated with a resonant molecular transition. In this technique, a single-frequency laser that is capable of being frequency chirped at high rates is used (e.g. diode laser, quantum cascade laser, VCSEL or wavelength-swept fiber laser). The Mach-Zehnder interferometer with an acousto-optical modulator (AOM) used as a frequency shifter are used to form a single, two-color laser beam. The two-color beam passes through a gas sample (enclosed in a gas cell or in open atmosphere) and then is focused onto a fast photodetector. When the laser frequency is chirped the molecular dispersion individually affects the propagation of both component waves leading to modulation of an instantaneous frequency of the heterodyne beatnote between the two waves. After frequency demodulation of the beatnote, information about the dispersion occurring in the optical path can be recovered and used for molecular concentration measurements.
Measurement of the frequency provides high immunity to laser power fluctuations (a similar effect is observed in conventional FM-demodulated radio signals). Moreover, in contrast to the absorption that becomes non-linear above ~10%, the measurement of the dispersion is linear within the entire range of sample concentrations and thus allows for large dynamic range of concentration measurements. CLaDS technique is suitable for any wavelength range and was characterized using mid-infrared distributed-feedback (DFB) quantum cascade lasers (QCLs) that give access to the strongest molecular ro-vibrational transitions. The linear dependence of the CLaDS signal on the molecular concentration as well as its immunity to the variations in the optical power reaching the photodetector were experimentally confirmed. Major noise contributions and their origins were identified and their individual impact on the observed signal-to-noise ratio (SNR) was estimated, which is used for optimization of CLaDS systems. One of the main limitations in conventional CLaDS is related to a quadratic dependence of the frequency demodulation noise with the acquisition bandwidth. Another limitation is a presence of a residual baseline in the measured spectrum due to a slight imbalance in the interferometer alignment. Since the frequency chirp is usually not perfectly linear the correction of such a residual baseline requires fitting by higher order polynomials, which affects quality of the retrieval. Therefore a new generation of CLaDS technology was developed to address those issues. A new detection scheme that involves chirp modulation technique provides all advantages of conventional CLaDS while eliminating both performance limitations.
CM-CLaDS
The principle of CM-CLaDS is based on a frequency chirp modulation that is obtained by applying sinusoidal modulation of the laser injection-current (similarly to wavelength modulation techniques used in standard laser absorption techniques). The CM-CLaDS can be used in two operating modes: 1) a spectral scan mode that uses a slow scan of the laser current to tune the laser or 2) the laser frequency can be permanently locked to the peak of the target molecular transition for continuous concentration monitoring. A CLaDS Signal that is recovered after frequency demodulation of the heterodyne beatnote is than post-processed in order to analyze its in-phase and quadrature components at the 1st and at the 2nd harmonic of the chirp modulation frequency. Such analysis of the CLaDS signal at higher (even) harmonics of the modulation frequency helps to overcome both fundamental limitations of the conventional CLaDS. First, an increase in SNR is obtained through significant reduction of the demodulation bandwidth resulting in lower demodulation noise. Second, since only odd harmonics in CM-CLaDS contain baseline originating from the imbalance of the interferometer arms, measurement of the 2nd harmonic CM-CLaDS signal enables truly baseline-free measurement. At the same time measurement of 1f component with a small (well controlled) interferometer imbalance enables determination of the instantaneous chirp rate of the laser. Since for most lasers the chirp rate varies while the wavelength is scanned, this capability provides a convenient way for a real-time chirp calibration with no need for any additional diagnostic instrumentation.
Experimental demonstration of the CM-CLaDS open-path remote sensing
To demonstrate capabilities of CM-CLaDS a prototype open-path remote sensing system was developed for molecular concentration measurements in ambient air (Fig. 1). With a distant retroreflector optical paths on the order of tens to thousands of meters can be obtained. Figure 1 shows two experimental CM-CLaDS dispersion spectra of nitrous oxide recorded around 4.55 μm at different detected powers. Despite a 20dB decrease in RF signal power the signal amplitudes and SNRs observed in both spectra are similar, which proves the immunity of CM-CLaDS to optical power variations. It is also clearly visible that both spectra are baseline-free (although there was a path difference between two interferometer arms). With 50 m optical path and the laser wavelength tuned to the center of the molecular transition at 2209.5 cm-1 the minimum detection limit of ~2 ppbv in 1 s was achieved. This corresponds to a bandwidth- and path-normalized N2O detection limit of ~100ppb⋅m/Hz1/2.
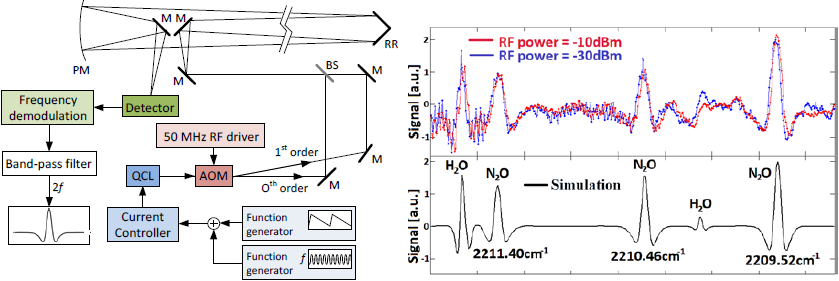
Fig. 1. Left panel: optical arrangement of the developed CM-CLaDS setup (RF ¿ radio frequency, M ¿ mirror, PM ¿ primary mirror, BS ¿ beam splitter, RR ¿ retroreflector); Right panel: dispersion spectra recorded using CM-CLaDS (top) and the HITRAN simulation (bottom). Despite being recorded at different powers of the heterodyne beatnote, both measured signals and SNRs are almost identical.
More details on CLaDS can be found in:
1) G. Wysocki and D. Weidmann, "Molecular dispersion spectroscopy for chemical sensing using chirped mid-infrared quantum cascade laser," Optics Express 18, 26123-26140 (2010).
2) M. Nikodem, C. Smith, D. Weidmann, G. Wysocki, "Remote mid-infrared sensing using chirped laser dispersion spectroscopy," Proceedings of SPIE Defense, Security, and Sensing 2011, paper 8024-14.
Contact: Renee Sanchez, Princeton University Office of Technology Licensing (609) 258-6762 renee.sanchez@princeton.edu